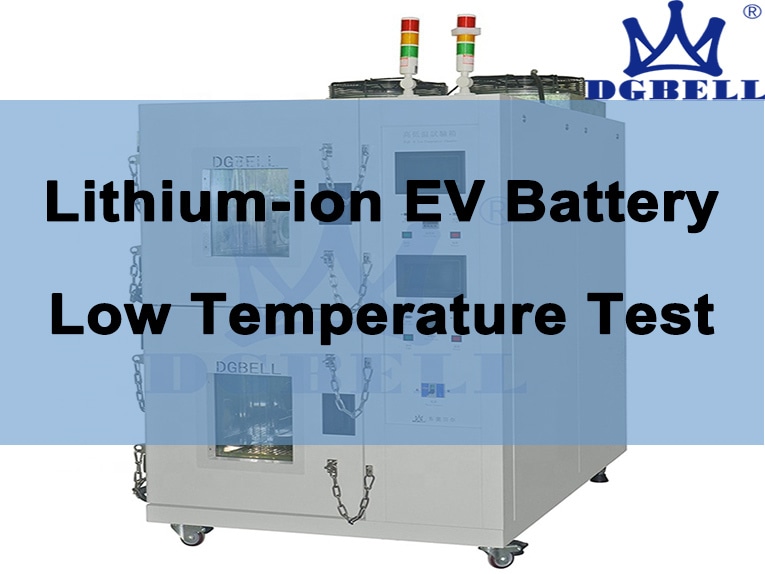
#Industry News
Lithium EV Battery Low Temperature Test
Lithium EV Battery Low Temperature Test
With the continuous development of power battery technology, lithium iron phosphate batteries are recognized as the most promising electrochemical power source due to their high safety, long cycle life, high energy density, and high voltage platform, and have been widely used in the field of electric vehicles. Power batteries are the direct source of energy for electric vehicles and a core component of electric vehicles. In the absence of significant breakthroughs in battery technology in a short period of time, exploring and improving the working performance of batteries as much as possible has become a key research direction.
Battery temperature is an important factor affecting the performance of power batteries. Lithium iron phosphate batteries have excellent performance at room temperature. When the lithium-ion battery operates below 0 ℃, its discharge performance will significantly deteriorate. As the charging and discharging cycle progresses, the battery capacity will undergo irreversible decay. In the study of the low-temperature characteristics and mechanism of lithium-ion batteries, too low a temperature can lead to the crystallization of liquid electrolytes, which slows down the migration rate of lithium ions between the positive and negative electrodes, resulting in poorer low-temperature charging and discharging characteristics of lithium-ion batteries.
In terms of low-temperature performance analysis of lithium-ion batteries, researchers at home and abroad have conducted extensive research, including first principles analysis, electrochemical impedance spectroscopy analysis, and charge discharge performance analysis. Establishing a thermal model for lithium iron phosphate batteries is an important method for studying their low-temperature characteristics. Currently, there are three main types of thermal models for lithium-ion batteries, namely the electrothermal coupling model, electrochemical thermal coupling model, and thermal abuse model.
This article focuses on the analysis of the low-temperature performance of lithium iron phosphate batteries and the simulation of battery thermal models, with a focus on the low-temperature performance of lithium iron phosphate power batteries. The aim is to systematically and comprehensively obtain the low-temperature characteristics of lithium iron phosphate batteries, providing a theoretical basis for their application in the field of electric vehicles.
1 Low temperature test and dynamic characteristics analysis
1.1 Test design for low-temperature performance of batteries
The experimental steps mainly include two parts: standard capacity testing at different temperatures and dynamic characteristic testing. The experimental steps can be summarized as follows:
(1) Select a brand new individual battery as the experimental object and place it in a temperature chamber. Set the temperature to room temperature of 25℃, let it stand for 2 hours, and use 1 C constant current discharge to discharge the battery to the cut-off voltage of 2.5 V
(2) Set the temperature of the incubator to the corresponding experimental temperature value, let it stand for 2 hours, and perform a standard capacity test on the battery. The standard capacity test includes two steps: constant current constant voltage charging (CCCV, constant current constant voltage, constant current 0.5 C, constant voltage 3.65 V, cut-off current 0.01 C), and 1 C constant current discharge with a cut-off voltage of 2.5 V. This step is used to obtain the charging and discharging capacity of the battery at the current ambient temperature;
(3) Set the temperature of the incubator to room temperature of 25 ℃, let it stand for 2 hours, and use the constant current constant voltage charging method (CCCV, constant current 0.5 C, constant voltage 3.65 V, cut-off current 0.01 C) to fully charge the battery
(4) To compare the performance of the battery at different temperatures, the above experimental steps were repeated at -15, 0, 10, 25, and 40 ℃, respectively.
A lithium-ion battery low-temperature performance testing experimental platform, specifically including a high and low temperature chamber for temperature control in the experimental environment: a battery testing system for battery performance testing; Lithium iron phosphate single cell battery, as the tested object; Upper computer software, used for custom battery testing method programming and battery testing data recording. The single cell battery used in the experiment is a soft pack lithium iron phosphate battery with a nominal capacity of 40 Ah.
1.2 Low temperature dynamic characteristics
Based on the experimental data of low-temperature performance of batteries, obtain the charging and discharging curves of lithium iron phosphate batteries at different temperatures. The battery charging adopts a constant current and constant voltage charging method. The charging current in the constant current stage is 0.5 C, and the charging voltage in the constant voltage stage is 3.65 V. When the charging current is less than 0.01 C, the charging stops; The battery discharge adopts 1 C constant current discharge, and stops when the battery terminal voltage drops to 2.50 V. It can be seen that the charging and discharging characteristics of a battery are greatly affected by temperature. The lower the temperature, the steeper the charging and discharging characteristics of the battery, and the smaller the available charging and discharging capacity of the battery.
Quantitative analysis of the charging and discharging curves of lithium iron phosphate batteries to obtain the available charging and discharging ranges for batteries at different temperatures. It can be seen that the lower the temperature, the smaller the available charging and discharging range of the battery. When the battery operates in an ambient temperature of -15 ℃, the available charging and discharging range of the battery only accounts for 22.2% of the nominal capacity, which can no longer meet the normal working requirements of the power battery. In addition, when the battery operates at 40 ℃, the measured maximum rechargeable limit of the battery can reach 110%. This is because the higher the temperature, the greater the Gibbs free energy of the electrochemical reaction in the battery, the greater the electrical work done, and the corresponding rechargeable capacity.
It should be noted that SOC is a relative quantity that needs to be defined in advance. This article defines it as follows: using the 0.01 C constant current discharge method, the battery is discharged to 2.50 V, and the state at the cut-off voltage is defined as 0% SOC; Using the constant current and constant voltage charging method (CCCV, constant current 0.5 C, constant voltage 3.65 V, cut-off current 0.01 C), the state of the battery when charged to the cut-off current 0.01 C is defined as 100% SOC; The above definitions are all conducted at room temperature of 25 ℃.
2 Parameter identification of thermal model
2.1 Identification of Battery Thermophysical Parameters
Based on the battery model, identify the model parameters through battery charging and discharging experiments. During the experiment, by placing the battery in an environment of 20 ℃, it can be approximately assumed that there is only natural convection heat transfer on the surface of the battery. Charging and discharging experiments were conducted at 1 C, 1.5 C, 2 C, and 2.5 C, with cut-off voltages of 3.65 and 250V, respectively. The charging reaction of a battery is an endothermic process, while the discharging reaction is an exothermic process. As the absolute values of the reaction heat during the charging and discharging processes are the same, only the discharging process is analyzed.
The curve graph of the temperature of the battery surface over time at different discharge rates. It can be seen that the temperature measurement values have small fluctuations, which are mainly caused by the high sensitivity of the thermal imager, multiple sampling points, and the influence of ambient light. These small fluctuations do not affect the overall trend of temperature changes. By using numerical methods to calculate the slopes of each curve, the slopes of the four curves can be fitted into a straight line, and according to the formula, the specific heat capacity of the battery C=-0.00333 can be calculated.
2.2 Identification of battery internal resistance
Due to the Joule heat caused by the internal resistance of the battery being the main heat source, in order to deeply analyze the Joule heat during the battery reaction process, it is necessary to identify the internal resistance values of the battery at different temperatures. Perform a Hybrid Pulse Power Characteristic Test (HPPC) on the battery every 10% SOC at experimental temperatures of -15, 0, 10, 25, and 40 ℃.
Based on test data, the least squares algorithm is used to identify the internal resistance of the battery at each test point. At different temperatures, the relationship curve between the internal resistance value of the battery and SOC was identified. It can be seen that the environmental temperature has a significant impact on the internal resistance of the battery, and the lower the environmental temperature, the greater the internal resistance of the battery.
3 Thermal model simulation
3.1 Verification of battery thermal model
In order to verify the accuracy of the model, it was validated through temperature experiments based on the battery thermal model structure and model parameters described above. The experiment was conducted at an ambient temperature of 24.8 ℃. During the experiment, the battery was discharged at a discharge rate of 0.5 C, and the temperature at the center of the battery surface was collected in real-time using a high-precision platinum resistance temperature sensor.
The battery thermal model has high accuracy and can simulate the actual heat generation reaction process of the battery. As the battery discharge reaction proceeds, the temperature at the center of the battery surface gradually increases. Due to the presence of natural convection heat transfer, the temperature at the center of the battery surface gradually reaches a stable trend over time.
3.2 Low temperature thermophysical characteristics
Simulated surface temperature distribution map of the battery when discharging at a rate of 0.5 C to a cut-off voltage of 2.5 V under different environmental temperatures. It can be seen that due to the heat generation effect during the electrochemical reaction process, the surface temperature of the battery is higher than the ambient temperature, and the minimum temperature of the battery surface also shows significant differences at different ambient temperatures. The reason for this phenomenon is that as the temperature decreases, the internal resistance of the battery increases in an approximate exponential pattern.
At lower ambient temperatures, the internal resistance of the battery significantly increases. When the same rate of discharge reaction occurs inside the battery, the phenomenon of heat generation due to internal resistance significantly increases. Therefore, according to the boundary conditions of convective heat transfer in batteries, the surface temperature of the battery will be higher than the ambient temperature.
At the same time, it can be seen that the temperature in the upper part of the battery, especially at the pole ear, has significantly increased compared to other parts, and there is a significant temperature gradient from the pole ear to the bottom of the battery. This is mainly determined by the thermal physical properties of the battery material. The battery cell is composed of various anisotropic materials, and the positive and negative electrode ears of the battery are made of metal aluminum and metal nickel, respectively. When the current flows through the electrode ears, the cross-sectional area significantly decreases, and the internal resistance increases sharply. The thermal effect of the internal resistance at the electrode ears is significantly higher than that of the battery core. Therefore, in the electrochemical reaction process of the battery, the vast majority of heat is generated at the electrode ears, and the heat is transferred from the electrode ears to the cell through thermal conduction, forming a clear temperature gradient.
4 Conclusion
This article investigates the low-temperature characteristics of lithium iron phosphate power batteries for electric vehicles. Firstly, low-temperature performance experiments of batteries were designed and conducted. The experimental results showed that the charging and discharging characteristics of batteries are greatly affected by temperature. The lower the temperature, the steeper the charging and discharging characteristics of batteries, and the smaller the available charging and discharging capacity of batteries. Secondly, a thermal model and low-temperature parameter identification method for lithium iron phosphate batteries were established. Finally, a simulation study was conducted on the thermal model of lithium iron phosphate batteries.
The simulation results show that the battery thermal model proposed in this paper has high accuracy and can simulate the actual heat generation reaction process of the battery. In the electrochemical reaction process of batteries, the vast majority of heat is generated at the electrode ears, and the heat is transferred from the electrode ears to the cell through thermal conduction, forming a significant temperature gradient. As the ambient temperature decreases, the temperature difference between the battery surface and the ambient temperature becomes larger, and the temperature gradient in the lower part of the battery surface will gradually increase.