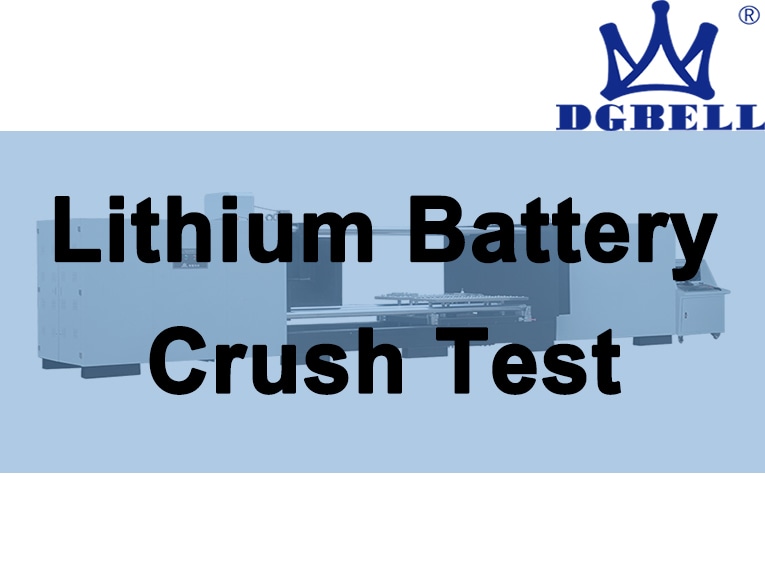
#Industry News
About Lithium Battery Crush Test
About Lithium Battery Crush Test
Due to limitations in materials, battery technology, and manufacturing processes, lithium-ion batteries have always faced significant fire risks during use. The safety of lithium-ion batteries under collision and compression has always been a focus of attention.
In general, fires caused by external forces in lithium-ion batteries are fundamentally caused by internal short circuits due to severe deformation and damage, resulting in intense electrochemical reactions and high heat generation inside the battery, ultimately leading to thermal runaway and combustion explosion of the battery.
Part of the experiments investigated the mechanical integrity of cylindrical lithium-ion batteries, and obtained the fault behavior of the batteries under different mechanical external forces through experiments and numerical simulations. Some researchers have studied the thermal runaway behavior of 18650 lithium-ion batteries under various crush methods. Even if the battery is not damaged or broken under compression, it may still develop into a catastrophic accident. Numerous research reports focus more on the fundamental theory of thermal runaway combustion in lithium-ion batteries, while there is relatively little research on the safety testing of lithium-ion battery crush.
At present, there are standards for the safety of lithium-ion batteries, which propose specific crush experimental methods for lithium-ion batteries and battery packs. The specified crush speed is (5 ± 1) mm/s, and the crush deformation is 30%. This is significantly different from the collision and compression conditions experienced by batteries in actual traffic accidents, and the evaluation of battery fire safety in the standards is also relatively vague. Only batteries that have truly burned or exploded are considered unqualified, and the fire hazard of batteries that have exploded or burned cannot be specifically evaluated. Therefore, it cannot meet the requirements of fire safety assessment, fire scene safety assessment, and fire investigation. The evaluation criteria of UL 1642 also stipulate that batteries are considered unqualified only if they experience a burning or explosion.
From this, it can be seen that the existing testing standards are only criteria for evaluating the qualification of battery products, and cannot be used to evaluate the fire hazard of batteries. The author of this article intends to conduct systematic compression experiments on common lithium-ion batteries to study the impact of different compression conditions on the fire safety of lithium-ion batteries, in order to provide a reference for the risk assessment of lithium-ion battery compression fire.
1 Test
1.1 Test object
18650 lithium-ion battery, SOC 50%
ICRIB1 lithium cobalt oxide battery
ICRIB2 lithium cobalt oxide battery
TCLIB3 nickel cobalt manganese lithium battery
1.2 Test methods
The experiment was conducted on the DGBELL BE-6047AP horizontal battery crush nail penetration testing machine. Fix the battery in the testing machine with a fixture so that the direction of the battery’s electrode plate is perpendicular to the direction of compression.
The four crush speeds used in the experiment were 50 mm/min, 100 mm/min, 200 mm/min, and 400 mm/min, respectively, with crush deformation variables of 10%, 20%, 30%, 40%, and 50%. Squeeze the battery at a set constant crush speed, stop squeezing when the battery deformation reaches the set value, and observe the battery.
During the experiment, the K-type thermal-couple of the temperature sensor was fixed on the positive electrode of the lithium-ion battery with high-temperature insulation tape. A customized temperature acquisition device was used to collect the temperature data measured by the thermal-couple and record the temperature changes of the positive electrode during the experiment.
2 Results and Discussion
2.1 The influence of crush deformation variables
When the crush speed is 50mm/min, record the temperature changes of the positive electrode surface of each battery over time in the crush experiment with different deformation variables.
From the experimental data, it can be seen that when the crush deformation is less than 20%, there is no significant change in the surface temperature of the positive electrode of ICRIB1 after compression. It is only after the crush deformation reaches 30% that the surface temperature of the positive electrode of ICRIB1 shows a significant increase, and the temperature rise rate accelerates. The temperature reaches its maximum value within 90 seconds, and then slowly decreases
The temperature change on the surface of the ICRIB2 positive electrode is similar to that of ICRIB1, and the temperature change is not significant when the crush deformation is lower than that of the ICRIB2 positive electrode surface. When the crush deformation is 30%, the extreme temperature of IRIB1 increases, with a maximum temperature of about 37 ° C. The exothermic time is longer, and the temperature decreases slowly.
When the crush deformation is 40%, the temperature of the positive electrode of the battery increases rapidly, reaching a maximum of about 57 ℃, and a large amount of electrolyte flows out from the positive electrode. When the deformation variable is set to 50% for crush, the temperature change trend of the positive electrode of the battery is similar to that of the deformation variable of 40%, with the highest temperature rising to about 63 ℃ and a large amount of electrolyte flowing out.
The temperature change on the surface of TCLIB3 positive electrode is significantly different from that of ICRIB1 and ICRIB2. When the crush deformation is below 10%, there is no significant change in the surface temperature of TCLIB3 positive electrode. When the crush deformation is 20%, the positive electrode temperature of TCLIB3 significantly increases, reaching a maximum temperature of about 47 ℃, and a small amount of electrolyte flows out.
When the crush deformation is 30% and 40%, the positive electrode temperature slightly increases, then slowly decreases. After releasing the crush head, the temperature rises again. When the crush deformation is 50%, the battery breaks after being squeezed, and the positive electrode temperature rises rapidly, reaching a maximum of about 95℃, then decreases. When the crush head is released, the temperature will rise again.
When the compression deformation of lithium cobalt oxide positive electrode batteries is within 20%, there is no obvious temperature abnormality. Only when the compression deformation is higher than 30%, it is easy to heat up and form a certain fire hazard. The ternary material positive electrode battery is sensitive to crush deformation changes. When the deformation is 20%, the positive electrode temperature significantly increases.
The highest positive electrode temperature in the crush experiment can reach about 95 ℃, which is higher than the 66 ℃ of the lithium cobalt oxide positive electrode battery. This indicates that the ternary material positive electrode battery has greater fire safety hazards when subjected to external compression. The compression results under different deformation variables indicate that for evaluating the fire safety of lithium-ion batteries in compression experiments, the deformation variable should be set to ≥ 30%.
2.2 Impact of crush speed
Set the deformation variable to 30% and observe the temperature variation of the positive electrode surface of each battery over time at different crush rates.
The surface temperature of the positive electrode of ICRIB1 rapidly increases after being squeezed, and a large amount of electrolyte can be observed flowing out during the experiment. When the crush speed is 100 mm/min, 200 mm/min, and 400 mm/min, the highest temperatures are about 68 ℃, 81 ℃, and 71 ℃, respectively. The crush speed has little effect on the temperature rise of ICRIB1, and the temperature rise of the positive electrode is basically the same for different crush speeds and the same crush deformation rate.
After increasing the battery capacity from 2.2 Ah of ICRIB1 to 2.6 Ah of ICRIB2, it becomes more sensitive to crush speed, and the surface temperature rise of the positive electrode further increases with the increase of crush speed. When the crush speed increases from 100 mm/min to 400 mm/min, the maximum surface temperature of the positive electrode increases from about 65 ° C to about 95 ° C, accompanied by a large amount of electrolyte flowing out.
The temperature change on the positive electrode surface of TCLIB3 is similar to that of ICRIB2, and the higher the crush speed, the higher the temperature rise. When the crush speed is 50 mm/min, 100 mm/min, and 400 mm/min, the highest temperatures are about 65 ℃, 78 ℃, and 106 ℃, respectively, which are higher than the highest temperature of ICRIB2 under the same experimental conditions. At the crush speed of 200 mm/min, TCLIB3 exploded, producing a large amount of white smoke, and the internal diaphragm and electrolyte sprayed out from the positive electrode. Due to damage to the positive electrode, complete temperature change data could not be collected.
The experimental results of different crush speeds indicate that the higher the crush speed, the higher the temperature rise of lithium-ion batteries, and the greater the fire hazard. Among the three types of lithium-ion batteries, the ternary material positive electrode battery exhibits the highest degree of temperature rise as the degree of compression increases, indicating the highest level of danger.
From the experimental comparison of different crush speeds, it can be seen that in the evaluation of fire safety of lithium-ion batteries, the crush speed should be set to ≥ 200 mm/min in order to better observe the differences between different lithium-ion batteries. At the same time, the temperature changes on the surface of lithium-ion batteries can serve as an important basis for judging fire safety, especially for lithium-ion batteries whose surface temperature can rise to over 100 ℃ during crush, there is a significant risk of natural combustion and explosion.
3 Conclusion
Lithium ion batteries are prone to internal short circuits under external compression, leading to ignition, combustion, and even explosion. To study the fire hazard of lithium-ion batteries under compression conditions, three types of lithium-ion batteries were selected to conduct compression ignition experiments under different compression deformation variables and compression speeds. The following conclusions were drawn:
When the crush deformation of lithium cobalt oxide positive electrode batteries is within 20%, there is no obvious abnormality in the battery temperature, and the danger is relatively low;
Only when the crush deformation reaches 30%, it is easy to generate heat and temperature, which poses a certain fire hazard.
When the deformation of the ternary material positive electrode battery is 20%, a significant temperature rise occurs, and the highest temperature reached is significantly higher than that of the lithium cobalt oxide positive electrode battery. When subjected to external compression, there is a greater fire safety hazard.
As the squeezing degree of lithium-ion batteries increases, the temperature rise also increases, and the risk of fire also increases.
Compared to lithium cobalt oxide positive electrode batteries, ternary material positive electrode batteries have a greater temperature rise with increasing crush speed, reaching higher temperatures, and are more prone to combustion and explosion.
When evaluating the fire safety of lithium-ion batteries through crush experiments, the deformation variable should be set to ≥ 30%, and the crush speed should be set to 200 mm/min. At the same time, the temperature change on the surface of lithium-ion batteries can serve as an important basis for judging fire safety.