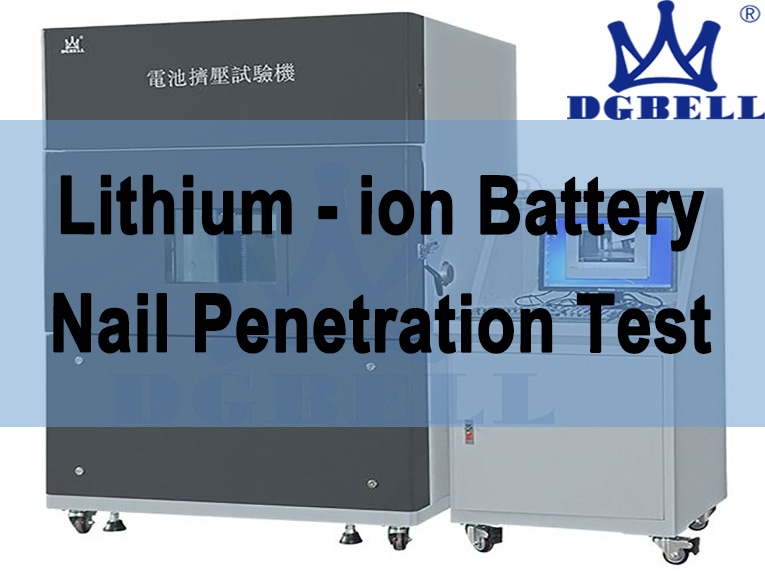
#Industry News
Lithium-ion Battery Nail Penetration Safety Test – Part 2
Lithium-ion Battery Nail Penetration Safety Test – Part 2
2.2 Different needle punching speeds
The loading speeds are set to 1 mm/min, 5 mm/min, 10 mm/min, and 20 mm/min.
It can be seen that the ultimate load increases with the increase of speed, and the maximum average ultimate load is 1.56 kN (20 mm/min). When the loading speed of the sample increases from 1mm/min to 20 mm/min, the average ultimate load increases by 14%.
Both experimental batteries in Group 2 experienced thermal runaway, with violent reactions occurring inside the batteries. A large amount of electrolyte flowed out from the puncture site and white smoke was emitted. From the voltage time curve, it can be seen that as the speed increases, the time required for voltage drop is significantly reduced, and the terminal voltage basically drops to around 0V.
The heating and cooling rates and peak temperatures of the battery after being penetrated at four different speeds are basically the same. This is because the membrane of the cylindrical battery has poor ductility, and it is easy to puncture the membrane during the needle piercing process, resulting in extremely short positive and negative terminals. The speed has little effect on the surface temperature of the selected battery in the experiment, and the peak temperature is slightly higher when the needle punching speed is 20 mm/min, reaching 100 ℃. Under other speed conditions, the peak temperature of the battery surface is basically the same, around 95 ℃, indicating that the energy released by the battery is less affected by the needle punching speed.
2.3 Different needle penetration depths
An increase in the depth of needle puncture will lead to an increase in the number of electrode points and contact area for short circuits, increasing the probability of direct short circuits. After short circuits, a series of side reactions will occur. Therefore, studying the impact of different depths on the safety performance of lithium batteries and finding the critical depth at which short circuits occur is of great significance for early warning.
When the depth is 10 mm, there is no change in voltage, indicating that there is no short circuit inside the lithium battery. When the depth is 11 mm, the voltage drops from 3.9 V to 3.6 V in about 124 seconds, then slowly rises to 3.78 V and remains at around 3.78 V, indicating that the positive and negative poles inside the battery have short circuited, causing a local short circuit.
Analyzing the reasons for voltage recovery, when the local heat at the acupuncture site is too high, the current collector, diaphragm, and local area of the puncture point may melt, thus cutting off the current path and hindering further discharge of the lithium battery. Whether the diaphragm and current collector melt together determines whether the voltage drop can be restored.
The peak temperature of the battery surface increases with the increase of penetration depth. When the needle depth is 10 mm and 11 mm, the surface temperature of the battery is not high, the degree of chemical reaction inside the battery is not severe, and no white smoke or irritating gas is emitted, indicating that thermal runaway has not occurred. When the lithium battery was punctured by 10mm, there was no short circuit and the temperature remained around 25 ℃.
When the needle depth is 11 mm, a local short circuit is triggered inside the battery, consuming a small amount of energy and resulting in a relatively slow heating rate.
When the steel needle penetrated to depths of 12 mm, 13 mm, and 16 mm, the battery experienced thermal runaway, emitting irritating gas and white smoke from the puncture site, and the electrolyte leaked. After piercing the shell, the temperature rises sharply and quickly reaches its peak, with the peak surface temperature of the battery exceeding 90 ℃.
In summary, it can be concluded that there is a critical depth from local short circuit to internal short circuit of the entire lithium battery when the steel needle penetrates the battery. The critical depth of the lithium battery used in this work is about 11 mm. When the puncture depth is less than the critical depth, the lithium battery will not experience thermal runaway, and the surface temperature of the battery will rise slowly. When the puncture depth exceeds the critical depth, the battery will experience thermal runaway and the surface temperature of the battery will rise sharply.
2.4 Different needle puncture positions
Three different locations were selected for the experiment
Different loading positions have a significant impact on the load-bearing capacity of the battery. The closer it is to the positive and negative terminals of the battery, the greater the ultimate load-bearing capacity and the greater the upward slope, and vice versa. There is a clear regularity in the influence of needle puncture position on the ultimate load of the battery. The closer the needle puncture position is to the axial center of the battery, the smaller the ultimate load, and vice versa, the greater the ultimate load.
The short circuit occurs first near the positive electrode and latest in the middle position. The voltage near the positive and negative poles did not drop sharply to 0 V after a sharp drop, but rebounded to higher voltages of 1.84 V and 1.1 V, and then began to fluctuate gradually to 0 V. This is because positions A and E are very close to the end of the battery core, and there is a gap between the end of the lithium battery core and the outer shell. During continuous loading, limited slip and stress concentration will occur, resulting in temporary voltage instability.
Under the same amount of deformation, the local strain at the edge position will be greater, leading to easier failure of the diaphragm and earlier occurrence of internal short circuits. Similarly, under the same amount of deformation, when the needle puncture position is close to the middle of the battery, the local strain will be smaller, so the internal short circuit will occur later.
The test all caused thermal runaway (a large amount of electrolyte flowed out from the needle puncture site and emitted a large amount of white smoke), and the closer the penetration location is to the positive and negative poles, the greater the risk of thermal runaway. The closer it is to the positive and negative terminals, the faster the battery heats up after a short circuit and the higher the peak temperature. Among them, the peak temperatures of the battery surface at positions 10 mm and 55 mm from the negative electrode are 110.3 ℃ and 104.8 ℃, respectively, and the peak temperature at position 30 mm from the negative electrode is 94.9 ℃. In summary, the edge position of the lithium battery selected in this work is more prone to thermal runaway.
3 Conclusion
The test used DGBELL Nail Penetration Test Chamber. A series of experiments were conducted on a single 18650 lithium-ion battery, including SOC, needle punching speed, depth, and position. The influence of different parameters on the safety performance of lithium batteries was analyzed using the force electric heat related data of batteries, and the following conclusions were drawn.
(1) The ultimate load of lithium batteries is not simply a relationship of increasing SOC. When the SOC is between 20% and 60%, the ultimate load increases with the increase of SOC; When the SOC is between 80% and 100%, it also increases with the increase of SOC, and the maximum average ultimate load is 1.66 kN, which is 20% higher than the average ultimate load of 20% SOC.
The larger the SOC, the more intense the reaction during the acupuncture process, and the greater the risk of thermal runaway. For different needling speeds, the rate of temperature rise and fall on the battery surface is consistent, with a peak temperature of around 95 ℃, and there is no significant correlation between needling speed and whether thermal runaway occurs.
(2) During the needling process, there is a critical depth from loca;l short circuit to causing a short circuit throughout the entire lithium battery. The critical depth of the lithium battery selected for the experiment is about 11 mm, which can be used as a threshold for early warning design to alert users.
Different positions have a significant impact on the force, electricity, and heat of lithium batteries. When the needle insertion position is close to the positive and negative electrodes, the ultimate load and peak temperature are higher than those in the middle position. The peak temperatures are 104.8 ℃ and 110.3 ℃, respectively. When lithium batteries are subjected to external force collisions near the positive and negative electrodes, their safety performance decreases, making them more prone to safety accidents such as thermal runaway.